Space-based solar power
![]() | This article possibly contains unsourced predictions, speculative material, or accounts of events that might not occur. Information must be verifiable and based on reliable published sources. (May 2016) |
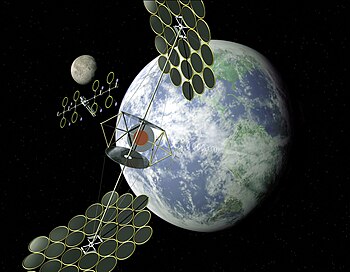
Part of a series on |
Sustainable energy |
---|
![]() |
|
Part of a series on |
Renewable energy |
---|
![]() |
Space-based solar power (SBSP, SSP) is the concept of collecting solar power in outer space with solar power satellites (SPS) and distributing it to Earth. Its advantages include a higher collection of energy due to the lack of reflection and absorption by the atmosphere, the possibility of very little night, and a better ability to orient to face the Sun. Space-based solar power systems convert sunlight to some other form of energy (such as microwaves) which can be transmitted through the atmosphere to receivers on the Earth's surface.
Various SBSP proposals have been researched since the early 1970s,<ref name="ESA-SBSP">"Space-based solar power". ESA–Advanced Concepts Team. 15 April 2013. Retrieved August 23, 2015.</ref><ref name="DOE-SBSP">"Space-Based Solar Power". United States Department of Energy (DOE). 6 March 2014.</ref> but none is economically viable with present-day space launch costs. Some technologists propose lowering launch costs with space manufacturing or with radical new space launch technologies other than rocketry.
Besides cost, SBSP also introduces several technological hurdles, including the problem of transmitting energy from orbit. Since wires extending from Earth's surface to an orbiting satellite are not feasible with current technology, SBSP designs generally include the wireless power transmission with its concomitant conversion inefficiencies, as well as land use concerns for antenna stations to receive the energy at Earth's surface. The collecting satellite would convert solar energy into electrical energy, power a microwave transmitter or laser emitter, and transmit this energy to a collector (or microwave rectenna) on Earth's surface. Contrary to appearances in fiction, most designs propose beam energy densities that are not harmful if human beings were to be inadvertently exposed, such as if a transmitting satellite's beam were to wander off-course. But the necessarily vast size of the receiving antennas would still require large blocks of land near the end users. The service life of space-based collectors in the face of long-term exposure to the space environment, including degradation from radiation and micrometeoroid damage, could also become a concern for SBSP.
As of 2020, SBSP is being actively pursued by Japan, China,<ref>Eric Rosenbaum; Donovan Russo (March 17, 2019). "China plans a solar power play in space that NASA abandoned decades ago". CNBC.com. Retrieved 19 March 2019.</ref> Russia, India, the United Kingdom,<ref>"UK government commissions space solar power stations research". gov.uk (Press release). UK Space Agency. 14 November 2020. Retrieved 30 November 2020.</ref> and the US.
In 2008, Japan passed its Basic Space Law which established space solar power as a national goal.<ref>"Basic Plan for Space Policy" (PDF). June 2, 2009. Retrieved May 21, 2016.</ref> JAXA has a roadmap to commercial SBSP.
In 2015, the China Academy for Space Technology (CAST) showcased its roadmap at the International Space Development Conference. In February 2019, Science and Technology Daily (科技日报, Keji Ribao), the official newspaper of the Ministry of Science and Technology of the People's Republic of China, reported that construction of a testing base had started in Chongqing's Bishan District. CAST vice-president Li Ming was quoted as saying China expects to be the first nation to build a working space solar power station with practical value. Chinese scientists were reported as planning to launch several small- and medium-sized space power stations between 2021 and 2025.<ref>"我国有望率先建成空间太阳能电站-科技新闻-中国科技网首页". www.stdaily.com. Retrieved 2021-08-18.</ref><ref>Needham, Kirsty (2019-02-15). "Plans for first Chinese solar power station in space revealed". The Sydney Morning Herald. Retrieved 2021-08-18.</ref> In December 2019, Xinhua News Agency reported that China plans to launch a 200-tonne SBSP station capable of generating megawatts (MW) of electricity to Earth by 2035.<ref>"China to build space-based solar power station by 2035 - Xinhua | English.news.cn". www.xinhuanet.com. Archived from the original on December 2, 2019. Retrieved 2021-08-18.</ref>
In May 2020, the US Naval Research Laboratory conducted its first test of solar power generation in a satellite.<ref>"Solar Power Experiment Launched by Navy Research Lab on X-37B Space Plane". Forbes. May 27, 2020.</ref> In August 2021, the California Institute of Technology (Caltech) announced that it planned to launch a SBSP test array by 2023, and at the same time revealed that Donald Bren and his wife Brigitte, both Caltech trustees, had been since 2013 funding the institute's Space-based Solar Power Project, donating over $100 million.<ref>"Caltech Announces Breakthrough $100 Million Gift to Fund Space-based Solar Power Project". California Institute of Technology. 3 August 2021. Retrieved 2021-08-18.</ref><ref name=caltech2023edu>"In a First, Caltech's Space Solar Power Demonstrator Wirelessly Transmits Power in Space". California Institute of Technology. 1 June 2023. Retrieved 2023-06-01.</ref> A Caltech team successfully demonstrated beaming power to earth in 2023.<ref name=caltech2023edu/>
History

In 1941, science fiction writer Isaac Asimov published the science fiction short story "Reason", in which a space station transmits energy collected from the Sun to various planets using microwave beams. The SBSP concept, originally known as satellite solar-power system (SSPS), was first described in November 1968.<ref>Glaser, P. E. (1968). "Power from the Sun: Its Future". Science. 162 (3856): 857–61. Bibcode:1968Sci...162..857G. doi:10.1126/science.162.3856.857. PMID 17769070.</ref> In 1973 Peter Glaser was granted U.S. patent number 3,781,647 for his method of transmitting power over long distances (e.g. from an SPS to Earth's surface) using microwaves from a very large antenna (up to one square kilometer) on the satellite to a much larger one, now known as a rectenna, on the ground.<ref name="Glaser" />
Glaser then was a vice president at Arthur D. Little, Inc. NASA signed a contract with ADL to lead four other companies in a broader study in 1974. They found that, while the concept had several major problems – chiefly the expense of putting the required materials in orbit and the lack of experience on projects of this scale in space – it showed enough promise to merit further investigation and research.<ref name="FS">Glaser, P. E., Maynard, O. E., Mockovciak, J., and Ralph, E. L, Arthur D. Little, Inc., "Feasibility study of a satellite solar power station", NASA CR-2357, NTIS N74-17784, February 1974</ref>
Concept Development and Evaluation Program
Between 1978 and 1986, the Congress authorized the Department of Energy (DoE) and NASA to jointly investigate the concept. They organized the Satellite Power System Concept Development and Evaluation Program.<ref>"Satellite Power System Concept Development and Evaluation Program July 1977 - August 1980. DOE/ET-0034, February 1978. 62 pages" (PDF). Archived from the original (PDF) on 2017-03-13. Retrieved 2009-02-20.</ref><ref>"Satellite Power System Concept Development and Evaluation Program Reference System Report. DOE/ER-0023, October 1978. 322" (PDF). Archived from the original (PDF) on 2017-03-13. Retrieved 2009-02-20.</ref> The study remains the most extensive performed to date (budget $50 million).<ref name="Mankins">Statement of John C. Mankins Archived 2014-04-19 at the Wayback Machine U.S. House Subcommittee on Space and Aeronautics Committee on Science, Sep 7, 2000</ref> Several reports were published investigating the engineering feasibility of such a project. They include:

- Resource Requirements (Critical Materials, Energy, and Land)<ref>"Satellite Power System (SPS) Resource Requirements (Critical Materials, Energy, and Land). HCP/R-4024-02, October 1978" (PDF). Archived from the original (PDF) on 2013-12-08. Retrieved 2009-02-20.</ref>
- Financial/Management Scenarios<ref>"Satellite Power System (SPS) Financial/Management Scenarios. Prepared by J. Peter Vajk. HCP/R-4024-03, October 1978. 69 pages" (PDF). Archived from the original (PDF) on 2013-12-08. Retrieved 2009-02-20.</ref><ref>"Satellite Power System (SPS) Financial/Management Scenarios. Prepared by Herbert E. Kierulff. HCP/R-4024-13, October 1978. 66 pages" (PDF). Archived from the original (PDF) on 2013-12-08. Retrieved 2009-02-20.</ref>
- Public Acceptance<ref>"Satellite Power System (SPS) Public Acceptance. HCP/R-4024-04, October 1978. 85 pages" (PDF). Archived from the original (PDF) on 2013-12-08. Retrieved 2009-02-20.</ref>
- State and Local Regulations as Applied to Satellite Power System Microwave Receiving Antenna Facilities<ref>"Satellite Power System (SPS) State and Local Regulations as Applied to Satellite Power System Microwave Receiving Antenna Facilities. HCP/R-4024-05, October 1978. 92 pages" (PDF). Archived from the original (PDF) on 2013-12-08. Retrieved 2009-02-20.</ref>
- Student Participation<ref>"Satellite Power System (SPS) Student Participation. HCP/R-4024-06, October 1978. 97 pages" (PDF). Archived from the original (PDF) on 2013-12-08. Retrieved 2009-02-20.</ref>
- Potential of Laser for SBSP Power Transmission<ref>"Potential of Laser for SPS Power Transmission. HCP/R-4024-07, October 1978. 112 pages" (PDF). Archived from the original (PDF) on 2013-12-08. Retrieved 2009-02-20.</ref>
- International Agreements<ref>"Satellite Power System (SPS) International Agreements. Prepared by Carl Q. Christol. HCP-R-4024-08, October 1978. 283 pages" (PDF). Archived from the original (PDF) on 2013-12-08. Retrieved 2009-02-20.</ref><ref>"Satellite Power System (SPS) International Agreements. Prepared by Stephen Grove. HCP/R-4024-12, October 1978. 86 pages" (PDF). Archived from the original (PDF) on 2013-12-08. Retrieved 2009-02-20.</ref>
- Centralization/Decentralization<ref>"Satellite Power System (SPS) Centralization/Decentralization. HCP/R-4024-09, October 1978. 67 pages" (PDF). Archived from the original (PDF) on 2013-12-08. Retrieved 2009-02-20.</ref>
- Mapping of Exclusion Areas For Rectenna Sites<ref>"Satellite Power System (SPS) Mapping of Exclusion Areas For Rectenna Sites. HCP-R-4024-10, October 1978. 117 pages" (PDF). Archived from the original (PDF) on 2014-02-24. Retrieved 2009-02-20.</ref>
- Economic and Demographic Issues Related to Deployment<ref>"Economic and Demographic Issues Related to Deployment of the Satellite Power System (SPS). ANL/EES-TM-23, October 1978. 71 pages" (PDF). Archived from the original (PDF) on 2013-12-08. Retrieved 2009-02-20.</ref>
- Some Questions and Answers<ref>"Some Questions and Answers About the Satellite Power System (SPS). DOE/ER-0049/1, January 1980. 47 pages" (PDF). Archived from the original (PDF) on 2013-12-08. Retrieved 2009-02-20.</ref>
- Meteorological Effects on Laser Beam Propagation and Direct Solar Pumped Lasers<ref>"Satellite Power Systems (SPS) Laser Studies: Meteorological Effects on Laser Beam Propagation and Direct Solar Pumped Lasers for the SPS. NASA Contractor Report 3347, November 1980. 143 pages" (PDF). Archived from the original (PDF) on 2013-12-08. Retrieved 2009-02-20.</ref>
- Public Outreach Experiment<ref>"Satellite Power System (SPS) Public Outreach Experiment. DOE/ER-10041-T11, December 1980. 67 pages" (PDF). Archived from the original (PDF) on 2013-12-08. Retrieved 2009-02-20.</ref>
- Power Transmission and Reception Technical Summary and Assessment<ref>http://www.nss.org/settlement/ssp/library/1981NASASPS-PowerTransmissionAndReception.pdf Archived 2013-12-08 at the Wayback Machine
"Satellite Power System Concept Development and Evaluation Program: Power Transmission and Reception Technical Summary and Assessment" NASA Reference Publication 1076, July 1981. 281 pages.</ref>
- Space Transportation<ref>"Satellite Power System Concept Development and Evaluation Program: Space Transportation. NASA Technical Memorandum 58238, November 1981. 260 pages" (PDF). Archived from the original (PDF) on 2013-12-08. Retrieved 2009-02-20.</ref>
Discontinuation
The project was not continued with the change in administrations after the 1980 United States elections. The Office of Technology Assessment concluded that "Too little is currently known about the technical, economic, and environmental aspects of SPS to make a sound decision whether to proceed with its development and deployment. In addition, without further research an SPS demonstration or systems-engineering verification program would be a high-risk venture."<ref>"Solar Power Satellites. Office of Technology Assessment, August 1981. 297 pages" (PDF). Archived from the original (PDF) on 2013-12-08. Retrieved 2009-02-20.</ref>
In 1997, NASA conducted its "Fresh Look" study to examine the modern state of SBSP feasibility. In assessing "What has changed" since the DOE study, NASA asserted that the "US National Space Policy now calls for NASA to make significant investments in technology (not a particular vehicle) to drive the costs of ETO [Earth to Orbit] transportation down dramatically. This is, of course, an absolute requirement of space solar power."<ref>"A Fresh Look at Space Solar Power: New Architectures, Concepts, and Technologies. John C. Mankins. International Astronautical Federation IAF-97-R.2.03. 12 pages" (PDF). Archived from the original (PDF) on 2017-10-26. Retrieved 2009-02-20.</ref>
Conversely, Pete Worden of NASA claimed that space-based solar is about five orders of magnitude more expensive than solar power from the Arizona desert, with a major cost being the transportation of materials to orbit. Worden referred to possible solutions as speculative and not available for decades at the earliest.<ref name="ReferenceA">"Dr. Pete Worden on thespaceshow". thespaceshow.com. 23 March 2009. Archived from the original on 7 July 2012.</ref>
On November 2, 2012, China proposed a space collaboration with India that mentioned SBSP, "may be Space-based Solar Power initiative so that both India and China can work for long term association with proper funding along with other willing space faring nations to bring space solar power to earth."<ref>"China proposes space collaboration with India". The Times of India. 2012-11-02. Archived from the original on 2013-05-23.</ref>
Exploratory Research and Technology program

In 1999, NASA initiated its Space Solar Power Exploratory Research and Technology program (SERT) for the following purposes:
- Perform design studies of selected flight demonstration concepts.
- Evaluate studies of the general feasibility, design, and requirements.
- Create conceptual designs of subsystems that make use of advanced SSP technologies to benefit future space or terrestrial applications.
- Formulate a preliminary plan of action for the U.S. (working with international partners) to undertake an aggressive technology initiative.
- Construct technology development and demonstration roadmaps for critical space solar power (SSP) elements.
SERT went about developing a solar power satellite (SPS) concept for a future gigawatt space power system, to provide electrical power by converting the Sun's energy and beaming it to Earth's surface, and provided a conceptual development path that would utilize current technologies. SERT proposed an inflatable photovoltaic gossamer structure with concentrator lenses or solar heat engines to convert sunlight into electricity. The program looked both at systems in Sun-synchronous orbit and geosynchronous orbit. Some of SERT's conclusions:
- The increasing global energy demand is likely to continue for many decades resulting in new power plants of all sizes being built.
- The environmental impact of those plants and their impact on world energy supplies and geopolitical relationships can be problematic.
- Renewable energy is a compelling approach, both philosophically and in engineering terms.
- Many renewable energy sources are limited in their ability to affordably provide the base load power required for global industrial development and prosperity, because of inherent land and water requirements.
- Based on their Concept Definition Study, space solar power concepts may be ready to reenter the discussion.
- Solar power satellites should no longer be envisioned as requiring unimaginably large initial investments in fixed infrastructure before the emplacement of productive power plants can begin.
- Space solar power systems appear to possess many significant environmental advantages when compared to alternative approaches.
- The economic viability of space solar power systems depends on many factors and the successful development of various new technologies (not least of which is the availability of much lower cost access to space than has been available); however, the same can be said of many other advanced power technologies options.
- Space solar power may well emerge as a serious candidate among the options for meeting the energy demands of the 21st century.<ref>Space Solar Power Satellite Technology Development at the Glenn Research Center—An Overview. James E. Dudenhoefer and Patrick J. George, NASA Glenn Research Center, Cleveland, Ohio.</ref>
- Launch costs in the range of $100–$200 per kilogram of payload from low Earth orbit to Geosynchronous orbit are needed if SPS is to be economically viable.<ref name="Mankins" />
Japan Aerospace Exploration Agency
The May 2014 IEEE Spectrum magazine carried a lengthy article "It's Always Sunny in Space" by Susumu Sasaki.<ref>"How Japan Plans to Build an Orbital Solar Farm". 24 April 2014.</ref> The article stated, "It's been the subject of many previous studies and the stuff of sci-fi for decades, but space-based solar power could at last become a reality—and within 25 years, according to a proposal from researchers at the Tokyo-based Japan Aerospace Exploration Agency (JAXA)."
JAXA announced on 12 March 2015 that they wirelessly beamed 1.8 kilowatts 50 meters to a small receiver by converting electricity to microwaves and then back to electricity. This is the standard plan for this type of power.<ref name="ATarantola">Tarantola, Andrew (12 March 2015). "Scientists make strides in beaming solar power from space" (PDF). Engadget. Vol. 162, no. 3856. pp. 857–861.</ref><ref name="PKT">"Japan space scientists make wireless energy breakthrough". www.thenews.com.pk.</ref> On 12 March 2015 Mitsubishi Heavy Industries demonstrated transmission of 10 kilowatts (kW) of power to a receiver unit located at a distance of 500 meters (m) away.<ref>"MHI Successfully Completes Ground Demonstration Testing of Wireless Power Transmission Technology for SSPS". 12 March 2015. Archived from the original on 15 March 2015. Retrieved 20 March 2015.</ref>
Advantages and disadvantages
Advantages
The SBSP concept is attractive because space has several major advantages over the Earth's surface for the collection of solar power:
- It is always solar noon in space and full sun.
- Collecting surfaces could receive much more intense sunlight, owing to the lack of obstructions such as atmospheric gasses, clouds, dust and other weather events. Consequently, the intensity in orbit is approximately 144% of the maximum attainable intensity on Earth's surface.[citation needed]
- A satellite could be illuminated over 99% of the time and be in Earth's shadow a maximum of only 72 minutes per night at the spring and fall equinoxes at local midnight.<ref>Solar Power Satellites. Washington, D.C.: Congress of the U.S., Office of Technology Assessment. August 1981. p. 66. LCCN 81600129.</ref> Orbiting satellites can be exposed to a consistently high degree of solar radiation, generally for 24 hours per day, whereas earth surface solar panels currently collect power for an average of 29% of the day.<ref name="ReferenceB">Collection at Earth's poles can take place for 24 hours per day, but there are very small loads demanded at the poles.</ref>
- Power could be relatively quickly redirected directly to areas that need it most. A collecting satellite could possibly direct power on demand to different surface locations based on geographical baseload or peak load power needs.
- Reduced plant and wildlife interference.
Disadvantages
The SBSP concept also has a number of problems:
- The large cost of launching a satellite into space. For 6.5 kg/kW, the cost to place a power satellite in geosynchronous orbit (GEO) cannot exceed $200/kg if the power cost is to be competitive.
- Microwave optic requires gigawatt scale to compensate for Airy disk beam spreading. Typically a 1 km transmitting disk at 2.45 GHz spreads out to 10 km at Earth distance.
- Inability to constrain power transmission inside tiny beam angles. For example, a beam of 0.002 degrees (7.2 arc seconds) is required to stay within a one kilometer receiving antenna target from geostationary altitude. The most advanced directional wireless power transfer systems as of 2019 spread their half power beam width across at least 0.9 arc degrees.<ref>Shen, G.; Liu, Y.; Sun, G.; Zheng, T.; Zhou, X.; Wang, A. (2019). "Suppressing Sidelobe Level of the Planar Antenna Array in Wireless Power Transmission". IEEE Access. 7: 6958–6970. doi:10.1109/ACCESS.2018.2890436. ISSN 2169-3536.</ref><ref>Wang, Wen-Qin (2019). "Retrodirective Frequency Diverse Array Focusing for Wireless Information and Power Transfer". IEEE Journal on Selected Areas in Communications. 37 (1): 61–73. doi:10.1109/JSAC.2018.2872360. ISSN 0733-8716. S2CID 56594774.</ref><ref>Shinohara, Naoki (June 2013). "Beam Control Technologies With a High-Efficiency Phased Array for Microwave Power Transmission in Japan". Proceedings of the IEEE. 101 (6): 1448–1463. doi:10.1109/JPROC.2013.2253062. hdl:2433/174333. S2CID 9091936.</ref><ref>Fartookzadeh, Mahdi (7 March 2019). "On the Time-Range Dependency of the Beampatterns Produced by Arbitrary Antenna Arrays: Discussions on the Misplaced Expectations from Frequency Diverse Arrays". arXiv:1903.03508 [physics.class-ph]. Bibcode:2019arXiv190303508F</ref>
- Inaccessibility: Maintenance of an earth-based solar panel is relatively simple, but construction and maintenance on a solar panel in space would typically be done telerobotically. In addition to cost, astronauts working in GEO are exposed to unacceptably high radiation dangers and risk and cost about one thousand times more than the same task done telerobotically.
- The space environment is hostile; PV panels (if used) suffer about eight times the degradation they would on Earth (except at orbits that are protected by the magnetosphere).<ref>In space, panels suffer rapid erosion from high energy particles,"Solar Panel Degradation" Archived 2011-09-29 at the Wayback Machine whereas on Earth, commercial panels degrade at a rate around 0.25% a year."Testing a Thirty-Year-Old Photovoltaic Module"</ref>
- Space debris is a major hazard to large objects in space, particularly for large structures such as SBSP systems in transit through the debris below 2000 km. Collision risk is much reduced in GEO since all the satellites are moving in the same direction at very close to the same speed.<ref>"Some of the most environmentally dangerous activities in space include [...] large structures such as those considered in the late-1970s for building solar power stations in Earth orbit."The Kessler Syndrome (As Discussed by Donald J. Kessler)". Retrieved 2010-05-26.</ref>
- The broadcast frequency of the microwave downlink (if used) would require isolating the SBSP systems away from other satellites. GEO space is already well used and would require coordinating with the ITU-R.<ref>Matsumoto, Hiroshi (2009). "Space Solar Power Satellite/Station and the Politics" (PDF). EMC'09/Kyoto. Archived from the original (PDF) on August 8, 2019. Retrieved August 7, 2021.</ref>
- The large size and corresponding cost of the receiving station on the ground. The cost has been estimated at a billion dollars for 5 GW by SBSP researcher Keith Henson.
- Energy losses during several phases of conversion from photons to electrons to photons back to electrons.<ref>"Elon Musk on SpaceX, Tesla, and More". Popular Mechanics. 2012-10-04. Retrieved 2023-06-15.</ref><ref>Swan, Philip (2019). "Wireless Power At-A-Distance Technology – A Strategy for Nurturing Ecosystem Development". 2019 IEEE PELS Workshop on Emerging Technologies: Wireless Power Transfer (WoW). IEEE. pp. 99–104. doi:10.1109/WoW45936.2019.9030683. ISBN 978-1-5386-7514-4. S2CID 212703930.
{{cite book}}
:|journal=
ignored (help)</ref> - Waste heat disposal in space power systems is difficult to begin with, but becomes intractable when the entire spacecraft is designed to absorb as much solar radiation as possible. Traditional spacecraft thermal control systems such as radiative vanes may interfere with solar panel occlusion or power transmitters.
- Decommissioning costs: The cost of deorbiting the satellites at the end of their service life to prevent them from exacerbating the orbital space debris problem due to impacts with asteroidal, cometary, and planetary debris<ref>Zolensky, Michael; Bland, Phil; Brown, Peter; Halliday, Ian (2006-07-01), "Flux of Extraterrestrial Materials", Meteorites and the Early Solar System II, University of Arizona Press, pp. 869–888, doi:10.2307/j.ctv1v7zdmm.46, retrieved 2023-06-15</ref> is likely to be significant. While the future cost of imparting Delta-V is difficult to estimate, the amount of Delta-V that must be imparted to transfer a satellite from GEO to GTO is 1472 m/s2. If, upon reentry, the disintegrating satellite would release hazardous chemicals into the Earth's atmosphere, then the additional expenses of disassembling the satellite and deorbiting the environmentally hazardous components within a space vehicle with downmass capabilities must be factored into the decommissioning costs.
Design

Space-based solar power essentially consists of three elements:<ref name="DOE-SBSP" />
- collecting solar energy in space with reflectors or inflatable mirrors onto solar cells or heaters for thermal systems
- wireless power transmission to Earth via microwave or laser
- receiving power on Earth via a rectenna, a microwave antenna
The space-based portion will not need to support itself against gravity (other than relatively weak tidal stresses). It needs no protection from terrestrial wind or weather, but will have to cope with space hazards such as micrometeors and solar flares. Two basic methods of conversion have been studied: photovoltaic (PV) and solar dynamic (SD). Most analyses of SBSP have focused on photovoltaic conversion using solar cells that directly convert sunlight into electricity. Solar dynamic uses mirrors to concentrate light on a boiler. The use of solar dynamic could reduce mass per watt. Wireless power transmission was proposed early on as a means to transfer energy from collection to the Earth's surface, using either microwave or laser radiation at a variety of frequencies.
Microwave power transmission
William C. Brown demonstrated in 1964, during Walter Cronkite's CBS News program, a microwave-powered model helicopter that received all the power it needed for flight from a microwave beam. Between 1969 and 1975, Bill Brown was technical director of a JPL Raytheon program that beamed 30 kW of power over a distance of 1 mile (1.6 km) at 9.6% efficiency.<ref>Dickenson, R.M. (1 September 1975). Evaluation of a Microwave High-Power Reception-Conversion Array for Wireless Power Transmission (JPL Technical Memorandum 33-741). NASA Jet Propulsion Laboratory. pp. 8–24. Retrieved 2 June 2019. Because of the small size of the array relative to the 26-m-diameter antenna tubular beam, only about 11.3% of the klystron transmitter output is incident on the array (see Fig. 12) and is thus available for collection and conversion to DC output.
</ref><ref name="Brown">Brown, W.C. (1984). "The History of Power Transmission by Radio Waves". IEEE Transactions on Microwave Theory and Techniques. 32 (9): 1230–1242. Bibcode:1984ITMTT..32.1230B. doi:10.1109/TMTT.1984.1132833. S2CID 73648082.</ref>
Microwave power transmission of tens of kilowatts has been well proven by existing tests at Goldstone in California (1975)<ref name="Brown"/><ref>"Wireless Power Transmission 34kw over 1 mile at 82.5% efficiency Goldstone 1975". 13 March 2008. Archived from the original on 2021-12-19 – via YouTube.</ref><ref>"Wireless Power Transmission for Solar Power Satellite (SPS) (Second Draft by N. Shinohara), Space Solar Power Workshop, Georgia Institute of Technology" (PDF).</ref> and Grand Bassin on Reunion Island (1997).<ref>POINT-TO-POINT WIRELESS POWER TRANSPORTATION IN REUNION ISLAND Archived 2005-10-23 at the Wayback Machine 48th International Astronautical Congress, Turin, Italy, 6–10 October 1997 – IAF-97-R.4.08 J. D. Lan Sun Luk, A. Celeste, P. Romanacce, L. Chane Kuang Sang, J. C. Gatina – University of La Réunion – Faculty of Science and Technology.</ref>

More recently, microwave power transmission has been demonstrated, in conjunction with solar energy capture, between a mountaintop in Maui and the island of Hawaii (92 miles away), by a team under John C. Mankins.<ref>POINT-TO-POINT WIRELESS POWER TRANSPORTATION IN HAWAII Archived 2010-06-20 at the Wayback Machine.</ref><ref>Researchers Beam 'Space' Solar Power in Hawaii by Loretta Hidalgo, September 12, 2008</ref> Technological challenges in terms of array layout, single radiation element design, and overall efficiency, as well as the associated theoretical limits are presently a subject of research, as it was demonstrated by the Special Session on "Analysis of Electromagnetic Wireless Systems for Solar Power Transmission" held during the 2010 IEEE Symposium on Antennas and Propagation.<ref>"2010 APS/URSI". July 26, 2009. Archived from the original on 2009-07-26.</ref> In 2013, a useful overview was published, covering technologies and issues associated with microwave power transmission from space to ground. It includes an introduction to SPS, current research and future prospects.<ref name= Sasaki>Sasaki, Susumu; Tanaka, Koji; Maki, Ken-Ichiro (2013). "Microwave Power Transmission Technologies for Solar Power Satellites". Proceedings of the IEEE. 101 (6): 1438. doi:10.1109/JPROC.2013.2246851. S2CID 23479022.</ref> Moreover, a review of current methodologies and technologies for the design of antenna arrays for microwave power transmission appeared in the Proceedings of the IEEE.<ref name= MASSA>Massa, Andrea; Oliveri, Giacomo; Viani, Federico; Rocca, Paolo (2013). "Array Designs for Long-Distance Wireless Power Transmission: State-of-the-Art and Innovative Solutions". Proceedings of the IEEE. 101 (6): 1464. doi:10.1109/JPROC.2013.2245491. S2CID 2990114.</ref>
Laser power beaming
Laser power beaming was envisioned by some at NASA as a stepping stone to further industrialization of space. In the 1980s, researchers at NASA worked on the potential use of lasers for space-to-space power beaming, focusing primarily on the development of a solar-powered laser. In 1989, it was suggested that power could also be usefully beamed by laser from Earth to space. In 1991, the SELENE project (SpacE Laser ENErgy) had begun, which included the study of laser power beaming for supplying power to a lunar base. The SELENE program was a two-year research effort, but the cost of taking the concept to operational status was too high, and the official project ended in 1993 before reaching a space-based demonstration.<ref>Glenn Involvement with Laser Power Beaming-- Overview Archived 2006-11-17 at the Wayback Machine NASA Glenn Research Center</ref>
Orbital location
The main advantage of locating a space power station in geostationary orbit is that the antenna geometry stays constant, and so keeping the antennas lined up is simpler. Another advantage is that nearly continuous power transmission is immediately available as soon as the first space power station is placed in orbit, LEO requires several satellites before they are producing nearly continuous power.
Power beaming from geostationary orbit by microwaves carries the difficulty that the required 'optical aperture' sizes are very large. For example, the 1978 NASA SPS study required a 1 km diameter transmitting antenna and a 10 km diameter receiving rectenna for a microwave beam at 2.45 GHz. These sizes can be somewhat decreased by using shorter wavelengths, although they have increased atmospheric absorption and even potential beam blockage by rain or water droplets. Because of the thinned array curse, it is not possible to make a narrower beam by combining the beams of several smaller satellites. The large size of the transmitting and receiving antennas means that the minimum practical power level for an SPS will necessarily be high; small SPS systems will be possible, but uneconomic.[original research?]
A collection of LEO (low earth orbit) space power stations has been proposed as a precursor to GEO (geostationary orbit) space-based solar power.<ref>Komerath, N.M; Boechler, N. (October 2006). The Space Power Grid. Valencia, Spain: 57th International Astronautical Federation Congress. IAC-C3.4.06. </ref>
Earth-based receiver
The Earth-based rectenna would likely consist of many short dipole antennas connected via diodes. Microwave broadcasts from the satellite would be received in the dipoles with about 85% efficiency.<ref>"CommSpacTransSec38.html". www.hq.nasa.gov.</ref> With a conventional microwave antenna, the reception efficiency is better, but its cost and complexity are also considerably greater. Rectennas would likely be several kilometers across.
In space applications
A laser SBSP could also power a base or vehicles on the surface of the Moon or Mars, saving on mass costs to land the power source. A spacecraft or another satellite could also be powered by the same means. In a 2012 report presented to NASA on space solar power, the author mentions another potential use for the technology behind space solar power could be for solar electric propulsion systems that could be used for interplanetary human exploration missions.<ref>Mankins, John. "SPS-ALPHA: The First Practical Solar Power Satellite via Arbitrarily Large Phased Array" (PDF). Archived from the original (PDF) on 23 May 2013. Retrieved 24 April 2014.</ref><ref>"Second Beamed Space-Power Workshop" (PDF). Nasa. 1989. pp. near page 290. Archived from the original (PDF) on 2012-04-02. Retrieved 2011-11-08.</ref><ref>Henry W. Brandhorst, Jr. (October 27, 2010). "Options for Lunar Power Beaming" (PDF). Brandhorst. FISO group. Archived from the original (PDF) on December 9, 2013. Retrieved January 5, 2012.</ref>
Launch costs
One problem with the SBSP concept is the cost of space launches and the amount of material that would need to be launched.
Much of the material launched need not be delivered to its eventual orbit immediately, which raises the possibility that high efficiency (but slower) engines could move SPS material from LEO to GEO at an acceptable cost. Examples include ion thrusters or nuclear propulsion.
To give an idea of the scale of the problem, assuming a solar panel mass of 20 kg per kilowatt (without considering the mass of the supporting structure, antenna, or any significant mass reduction of any focusing mirrors) a 4 GW power station would weigh about 80,000 metric tons,<ref>"Space-Based Solar Power". energy.gov.</ref> all of which would, in current circumstances, be launched from the Earth. This is, however, far from the state of the art for flown spacecraft, which as of 2015 was 150 W/kg (6.7 kg/kW), and improving rapidly.<ref>"Solar Power and Energy Storage for Planetary Missions" (PDF). August 25, 2015.</ref> Very lightweight designs could likely achieve 1 kg/kW,<ref>"Case For Space Based Solar Power Development". August 2003. Retrieved 2006-03-14.</ref> meaning 4,000 metric tons for the solar panels for the same 4 GW capacity station. Beyond the mass of the panels, overhead (including boosting to the desired orbit and stationkeeping) must be added.
1 kg/kW | 5 kg/kW | 20 kg/kW | |
---|---|---|---|
$1/kg (Minimum cost at ~$0.13/kWh power, 100% efficiency) | $4M | $20M | $80M |
$2000/kg (ex: Falcon Heavy) | $8B | $40B | $160B |
$10000/kg (ex: Ariane V) | $40B | $200B | $800B |
To these costs must be added the environmental impact of heavy space launch missions, if such costs are to be used in comparison to earth-based energy production. For comparison, the direct cost of a new coal<ref>"2006_program_update" (PDF). Archived from the original (PDF) on 2007-01-10.</ref> or nuclear power plant ranges from $3 billion to $6 billion per GW (not including the full cost to the environment from CO2 emissions or storage of spent nuclear fuel, respectively).
Building from space
From lunar materials launched in orbit
Gerard O'Neill, noting the problem of high launch costs in the early 1970s, proposed building the SPS's in orbit with materials from the Moon.<ref>O'Neill, Gerard K., "The High Frontier, Human Colonies in Space", ISBN 0-688-03133-1, P.57</ref> Launch costs from the Moon are potentially much lower than from Earth because of the lower gravity and lack of atmospheric drag. This 1970s proposal assumed the then-advertised future launch costing of NASA's space shuttle. This approach would require substantial upfront capital investment to establish mass drivers on the Moon.<ref>"Colonizing Space - '70s Style!". 11 December 2009. Archived from the original on 2021-12-19 – via YouTube.</ref> Nevertheless, on 30 April 1979, the Final Report ("Lunar Resources Utilization for Space Construction") by General Dynamics' Convair Division, under NASA contract NAS9-15560, concluded that use of lunar resources would be cheaper than Earth-based materials for a system of as few as thirty solar power satellites of 10 GW capacity each.<ref>General Dynamics Convair Division (1979). Lunar Resources Utilization for Space Construction (PDF). GDC-ASP79-001.</ref>
In 1980, when it became obvious NASA's launch cost estimates for the space shuttle were grossly optimistic, O'Neill et al. published another route to manufacturing using lunar materials with much lower startup costs.<ref>O'Neill, Gerard K.; Driggers, G.; O'Leary, B. (1980). "New Routes to Manufacturing in Space". Astronautics and Aeronautics. 18: 46–51. Bibcode:1980AsAer..18...46G. Several scenarios for the buildup of industry in space are described. One scenario involves a manufacturing facility, with a crew of three, entirely on the lunar surface. Another scenario involves a fully automated manufacturing facility, remotely supervised from the earth, with provision for occasional visits by repair crews. A third case involves a crewed facility on the Moon for operating a mass-driver launcher to transport lunar materials to a collection point in space and for replicating mass-drivers.</ref> This 1980s SPS concept relied less on human presence in space and more on partially self-replicating systems on the lunar surface under remote control of workers stationed on Earth. The high net energy gain of this proposal derives from the Moon's much shallower gravitational well.
Having a relatively cheap per pound source of raw materials from space would lessen the concern for low mass designs and result in a different sort of SPS being built. The low cost per pound of lunar materials in O'Neill's vision would be supported by using lunar material to manufacture more facilities in orbit than just solar power satellites. Advanced techniques for launching from the Moon may reduce the cost of building a solar power satellite from lunar materials. Some proposed techniques include the lunar mass driver and the lunar space elevator, first described by Jerome Pearson.<ref>Pearson, Jerome; Eugene Levin, John Oldson and Harry Wykes (2005). Lunar Space Elevators for Cislunar Space Development Phase I Final Technical Report (PDF).</ref> It would require establishing silicon mining and solar cell manufacturing facilities on the Moon.[citation needed]
On the Moon
Physicist Dr David Criswell suggests the Moon is the optimum location for solar power stations, and promotes lunar-based solar power.<ref>"UH Mobile - Space-Related Centers at UH Target Next 50 Years of Exploration".</ref><ref>"Criswell - Publications and Abstracts". Archived from the original on 2010-06-22.</ref><ref>David Warmflash (29 March 2017). "Beaming solar energy from the Moon could solve Earth's energy crisis". Wired UK. Condé Nast. Retrieved February 27, 2018.</ref> The main advantage he envisions is construction largely from locally available lunar materials, using in-situ resource utilization, with a teleoperated mobile factory and crane to assemble the microwave reflectors, and rovers to assemble and pave solar cells,<ref>"Lunar Solar Cell Manufacturing" (PDF). www.cam.uh.edu. Archived from the original (PDF) on 22 June 2010. Retrieved 12 January 2022.</ref> which would significantly reduce launch costs compared to SBSP designs. Power relay satellites orbiting around earth and the Moon reflecting the microwave beam are also part of the project. A demo project of 1 GW starts at $50 billion.<ref>DAVID R. CRISWEL. LUNAR SOLAR POWER SYSTEM: INDUSTRIAL RESEARCH, DEVELOPMENT, AND DEMONSTRATION (PDF). 18th Congress, Buenos Aires, October 2001. World Energy Council. Archived from the original (PDF) on 26 March 2012. Retrieved 12 January 2022 – via www.moonbase-italia.org.</ref> The Shimizu Corporation use combination of lasers and microwave for the Luna Ring concept, along with power relay satellites.<ref>"The Luna Ring concept". Solar System Exploration Research Virtual Institute.</ref><ref>"Lunar Solar Power Generation, "The LUNA RING", Concept and Technology" (PDF). Japan-U.S. Science, Technology & Space Applications Program. 2009. Archived from the original (PDF) on 2013-12-08.</ref>
From an asteroid
Asteroid mining has also been seriously considered. A NASA design study<ref>Space Resources, NASA SP-509, Vol 1.</ref> evaluated a 10,000-ton mining vehicle (to be assembled in orbit) that would return a 500,000-ton asteroid fragment to geostationary orbit. Only about 3,000 tons of the mining ship would be traditional aerospace-grade payload. The rest would be reaction mass for the mass-driver engine, which could be arranged to be the spent rocket stages used to launch the payload. Assuming that 100% of the returned asteroid was useful, and that the asteroid miner itself couldn't be reused, that represents nearly a 95% reduction in launch costs. However, the true merits of such a method would depend on a thorough mineral survey of the candidate asteroids; thus far, we have only estimates of their composition.<ref>"Retrieval of Asteroidal Materials". Archived from the original on 2010-05-31.</ref> One proposal is to capture the asteroid Apophis into Earth orbit and convert it into 150 solar power satellites of 5 GW each or the larger asteroid 1999 AN10, which is 50 times the size of Apophis and large enough to build 7,500 5-gigawatt solar power satellites<ref>Stephen D. Covey (May 2011). "Technologies for Asteroid Capture into Earth Orbit". Archived from the original on 2011-12-12. Retrieved 2012-01-29.</ref>
Gallery
-
A Lunar base with a mass driver (the long structure that goes toward the horizon). NASA conceptual illustration
-
An artist's conception of a "self-growing" robotic lunar factory.
-
Microwave reflectors on the moon and teleoperated robotic paving rover and crane.
-
"Crawler" traverses Lunar surface, smoothing, melting a top layer of regolith, then depositing elements of silicon PV cells directly on surface
-
Sketch of the Lunar Crawler to be used for fabrication of lunar solar cells on the surface of the Moon.
-
Shown here is an array of solar collectors that convert power into microwave beams directed toward Earth.
-
A solar power satellite built from a mined asteroid.
Safety
The use of microwave transmission of power has been the most controversial issue in considering any SPS design. At the Earth's surface, a suggested microwave beam would have a maximum intensity at its center, of 23 mW/cm2 (less than 1/4 the solar irradiation constant), and an intensity of less than 1 mW/cm2 outside the rectenna fenceline (the receiver's perimeter).<ref name="intensity">Hanley., G.M.. . "Satellite Concept Power Systems (SPS) Definition Study" (PDF). NASA CR 3317, Sept 1980. </ref> These compare with current United States Occupational Safety and Health Act (OSHA) workplace exposure limits for microwaves, which are 10 mW/cm2,<ref>Radiofrequency and Microwave Radiation Standards interpretation of General Industry (29 CFR 1910) 1910 Subpart G, Occupational Health and Environmental Control 1910.97, Non-ionizing radiation.</ref>[original research?] - the limit itself being expressed in voluntary terms and ruled unenforceable for Federal OSHA enforcement purposes.[citation needed] A beam of this intensity is therefore at its center, of a similar magnitude to current safe workplace levels, even for long term or indefinite exposure.[original research?] Outside the receiver, it is far less than the OSHA long-term levels<ref>2081 A Hopeful View of the Human Future, by Gerard K. O'Neill, ISBN 0-671-24257-1, P. 182-183</ref> Over 95% of the beam energy will fall on the rectenna. The remaining microwave energy will be absorbed and dispersed well within standards currently imposed upon microwave emissions around the world.<ref>Griffin, D. (1983). "A microwave antenna method of measuring the effect of metal-framed spectacles on microwaves near the eyes". 1983 Antennas and Propagation Society International Symposium. Vol. 21. pp. 253–256. doi:10.1109/APS.1983.1149129.</ref> It is important for system efficiency that as much of the microwave radiation as possible be focused on the rectenna. Outside the rectenna, microwave intensities rapidly decrease, so nearby towns or other human activity should be completely unaffected.<ref name="IEEE 602864"/>
Exposure to the beam can be minimized in other ways. On the ground, physical access is controllable (e.g., via fencing), and typical aircraft flying through the beam provide passengers with a protective metal shell (i.e., a Faraday Cage), which will intercept the microwaves. Other aircraft (balloons, ultralight, etc.) can avoid exposure by using controlled airspace, as is currently done for military and other controlled airspace. The microwave beam intensity at ground level in the center of the beam would be designed and physically built into the system; simply, the transmitter would be too far away and too small to be able to increase the intensity to unsafe levels, even in principle.
In addition, a design constraint is that the microwave beam must not be so intense as to injure wildlife, particularly birds. Experiments with deliberate microwave irradiation at reasonable levels have failed to show negative effects even over multiple generations.<ref>"Beam Effects". www.permanent.com. Archived from the original on 2019-06-08. Retrieved 2021-10-21.</ref> Suggestions have been made to locate rectennas offshore,<ref>"Solar power satellite offshore rectenna study" (PDF). Final Report Rice Univ. 1980. Bibcode:1980ruht.reptT.....</ref><ref>Freeman, J. W.; et al. (1980). "Offshore rectenna feasibility". In NASA, Washington the Final Proc. of the Solar Power Satellite Program Rev. P 348-351 (SEE N82-22676 13-44): 348. Bibcode:1980spsp.nasa..348F. hdl:2060/19820014867.</ref> but this presents serious problems, including corrosion, mechanical stresses, and biological contamination.
A commonly proposed approach to ensuring fail-safe beam targeting is to use a retrodirective phased array antenna/rectenna. A "pilot" microwave beam emitted from the center of the rectenna on the ground establishes a phase front at the transmitting antenna. There, circuits in each of the antenna's subarrays compare the pilot beam's phase front with an internal clock phase to control the phase of the outgoing signal. This forces the transmitted beam to be centered precisely on the rectenna and to have a high degree of phase uniformity; if the pilot beam is lost for any reason (if the transmitting antenna is turned away from the rectenna, for example) the phase control value fails and the microwave power beam is automatically defocused.<ref name="IEEE 602864">Gupta, S.; Fusco, V.F. (1997). "Automatic beam steered active antenna receiver". 1997 IEEE MTT-S International Microwave Symposium Digest. Vol. 2. pp. 599–602. doi:10.1109/MWSYM.1997.602864. ISBN 978-0-7803-3814-2. S2CID 21796252.</ref> Such a system would be physically incapable of focusing its power beam anywhere that did not have a pilot beam transmitter. The long-term effects of beaming power through the ionosphere in the form of microwaves has yet to be studied, but nothing has been suggested which might lead to any significant effect.
Timeline
In the 20th century
- 1941: Isaac Asimov published the science fiction short story "Reason," in which a space station transmits energy collected from the sun to various planets using microwave beams. "Reason" was published in the "Astounding Science Fiction" magazine. <ref>"Reason". Goodreads. Retrieved 2024-02-29.</ref>
- 1968: Peter Glaser introduces the concept of a "solar power satellite" system with square miles of solar collectors in high geosynchronous orbit for collection and conversion of sun's energy into a microwave beam to transmit usable energy to large receiving antennas (rectennas) on Earth for distribution.
- 1973: Peter Glaser is granted United States patent number 3,781,647 for his method of transmitting power over long distances using microwaves from a large (one square kilometer) antenna on the satellite to a much larger one on the ground, now known as a rectenna.<ref name="Glaser">Glaser, Peter E. (December 25, 1973). "Method And Apparatus For Converting Solar Radiation To Electrical Power". United States Patent 3,781,647.</ref>
- 1978–1981: The United States Department of Energy and NASA examine the solar power satellite (SPS) concept extensively, publishing design and feasibility studies.
- 1987: Stationary High Altitude Relay Platform a Canadian experiment
- 1995–1997: NASA conducts a "Fresh Look" study of space solar power (SSP) concepts and technologies.
- 1998: The Space Solar Power Concept Definition Study (CDS) identifies credible, commercially viable SSP concepts, while pointing out technical and programmatic risks.
- 1998: Japan's space agency begins developing a space solar power system (SSPS), a program that continues to the present day.<ref>"Introduction of Research: About the SSPS". JAXA. Retrieved 25 November 2022.</ref>
- 1999: NASA's Space Solar Power Exploratory Research and Technology program (SERT, see below) begins.
- 2000: John Mankins of NASA testifies in the U.S. House of Representatives, saying "Large-scale SSP is a very complex integrated system of systems that requires numerous significant advances in current technology and capabilities. A technology roadmap has been developed that lays out potential paths for achieving all needed advances — albeit over several decades.<ref name="Mankins" />
In the 21st century
- 2001: NASDA (One of Japan's national space agencies before it became part of JAXA) announces plans to perform additional research and prototyping by launching an experimental satellite with 10 kilowatts and 1 megawatt of power.<ref>"Controversy Flares Over Space-Based Solar Power Plans". Space.com. 2 December 2009.</ref><ref>Presentation of relevant technical background with diagrams: http://www.spacefuture.com/archive/conceptual_study_of_a_solar_power_satellite_sps_2000.shtml</ref>
- 2003: ESA studies<ref>"History of research on SPS". Archived from the original on 2012-10-22.</ref>
- 2007: The US Pentagon's National Security Space Office (NSSO) issues a report<ref>"National Security Space Office Interim Assessment Phase 0 Architecture Feasibility Study, October 10, 2007" (PDF). Archived from the original (PDF) on October 25, 2007. Retrieved October 20, 2007.</ref> on October 10, 2007 stating they intend to collect solar energy from space for use on Earth to help the United States' ongoing relationship with the Middle East and the battle for oil. A demo plant could cost $10 billion, produce 10 megawatts, and become operational in 10 years.<ref>"Making the case, again, for space-based solar power". thespacereview.com. November 28, 2011.</ref>
- 2007: In May 2007, a workshop is held at the US Massachusetts Institute of Technology (MIT) to review the current state of the SBSP market and technology.<ref>Terrestrial Energy Generation Based on Space Solar Power: A Feasible Concept or Fantasy? Date: May 14–16, 2007; Location: MIT, Cambridge MA</ref>
- 2010: Professors Andrea Massa and Giorgio Franceschetti announce a special session on the "Analysis of Electromagnetic Wireless Systems for Solar Power Transmission" at the 2010 Institute of Electrical and Electronics Engineers International Symposium on Antennas and Propagation.<ref>Special Session list, IEEE International Symposium on Antennas and Propagation, April 20, 2010</ref>
- 2010: The Indian Space Research Organisation and US' National Space Society launched a joint forum to enhance partnership in harnessing solar energy through space-based solar collectors. Called the Kalam-NSS Initiative after the former Indian President Dr APJ Abdul Kalam, the forum will lay the groundwork for the space-based solar power program which could see other countries joining in as well.<ref>Mridul Chadha (November 10, 2010), US, India launch space based solar energy initiative, archived from the original on July 31, 2012</ref>
- 2010: Sky's No Limit: Space-Based solar power, the next major step in the Indo-US strategic partnership? written by USAF Lt Col Peter Garretson was published at the Institute for Defence Studies and Analysis.<ref>"Sky's No Limit: Space-based solar power, the next major step in the Indo-US strategic partnership? | Institute for Defence Studies and Analyses". www.idsa.in. Retrieved 2016-05-21.</ref>
- 2012: China proposed joint development between India and China towards developing a solar power satellite, during a visit by former Indian President Dr APJ Abdul Kalam.<ref>PTI (November 2, 2012), "US, China proposes space collaboration with India", The Times of India, archived from the original on May 23, 2013</ref>
- 2015: The Space Solar Power Initiative (SSPI) is established between Caltech and Northrop Grumman Corporation. An estimated $17.5 million is to be provided over a three-year project for development of a space-based solar power system.
- 2015: JAXA announced on 12 March 2015 that they wirelessly beamed 1.8 kilowatts 50 meters to a small receiver by converting electricity to microwaves and then back to electricity.<ref name="ATarantola" /><ref name="PKT" />
- 2016: Lt Gen. Zhang Yulin, deputy chief of the [PLA] armament development department of the Central Military Commission, suggested that China would next begin to exploit Earth-Moon space for industrial development. The goal would be the construction of space-based solar power satellites that would beam energy back to Earth.<ref>"Exploiting earth-moon space: China's ambition after space station". Xinhua News Agency. Archived from the original on March 8, 2016. Retrieved 2016-05-21.</ref>
- 2016: A team with membership from the Naval Research Laboratory (NRL), Defense Advanced Projects Agency (DARPA), Air Force Air University, Joint Staff Logistics (J-4), Department of State, Makins Aerospace and Northrop Grumman won the Secretary of Defense (SECDEF) / Secretary of State (SECSTATE) / USAID Director's agency-wide D3 (Diplomacy, Development, Defense) Innovation Challenge with a proposal that the US must lead in space solar power. The proposal was followed by a vision video
- 2016: Citizens for Space-Based Solar Power has transformed the D3 proposal into active petitions on the White House Website "America Must Lead the Transition to Space-Based Energy"and Change.org "USA Must Lead the Transition to Space-Based Energy" along with the following video.
- 2016: Erik Larson and others from NOAA produce a paper "Global atmospheric response to emissions from a proposed reusable space launch system"<ref>Larson, Erik J. L.; Portmann, Robert W.; Rosenlof, Karen H.; Fahey, David W.; Daniel, John S.; Ross, Martin N. (2017). "Global atmospheric response to emissions from a proposed reusable space launch system". Earth's Future. 5 (1): 37–48. Bibcode:2017EaFut...5...37L. doi:10.1002/2016EF000399.</ref> The paper makes a case that up to 2 TW/year of power satellites could be constructed without intolerable damage to the atmosphere. Before this paper, there was concern that the NOx produced by reentry would destroy too much ozone.
- 2016: Ian Cash of SICA Design proposes CASSIOPeiA (Constant Aperture, Solid State, Integrated, Orbital Phased Array) a new concept SPS Faculty Listing | Electrical and Computer Engineering
- 2017: NASA selects five new research proposals focused on investments in space. The Colorado School of Mines focuses on "21st Century Trends in Space-Based Solar Power Generation and Storage."
- 2019: Aditya Baraskar and Prof Toshiya Hanada from Space System Dynamic Laboratory, Kyushu University proposed Energy Orbit (E-Orbit),<ref name="E-Orbit">"Energy Orbit". 6th Space Solar Power (SSPS) Symposium (Online). 4 December 2020.</ref> a small Space Solar Power Satellite constellation for power beaming between satellites in low earth orbit. A total of 1600 satellites to transmit 10 kilowatts of electricity in a 500 km radius at an altitude of 900 km.<ref name="APRSAF26">"Satellite to Satellite Wireless Power Transmission System for small Space Solar Power Station". The 26th Session of the Asia-Pacific Regional Space Agency Forum (APRSAF-26). 26 November 2019.</ref>
- 2019: China creates a test base for SBSP, and announces plan to launch a working megawatt-grade 200-tonne SBSP station by 2035.
- 2020: US Naval Research Laboratory launches test satellite.<ref name="usnavy2020">"Naval Research Laboratory Conducts First Test of Solar Power Satellite Hardware in Orbit". www.navy.mil (Press release). U.S. Naval Research Laboratory Public Affairs. May 18, 2020. Archived from the original on May 19, 2020. Retrieved 19 May 2020.</ref> Also the USAF has its Space Solar Power Incremental Demonstrations and Research Project (SSPIDR) planning to launch the ARACHNE test satellite.<ref>"SPACE POWER BEAMING – Air Force Research Laboratory". Archived from the original on 2021-04-28. Retrieved 2021-04-28.</ref> Arachne is due to launch in 2024.<ref>David, Leonard (April 8, 2021). "Space-based solar power getting key test aboard US military's mysterious X-37B space plane". Space.com.</ref>
- 2021: Caltech announces that it planned to launch a SBSP test array by 2023.
- 2022: The Space Energy Initiative in the UK announced to launch the first power station in space during the mid-2040s, to "provide 30 percent of the UK’s (greatly increased) electricity demand" and "to slash the UK’s dependence on fossil fuels" and foreign ties.<ref>"UK to launch first power station in SPACE – limitless green energy to slash foreign ties". Space Energy Initiative. 21 March 2022. Retrieved 18 April 2022.</ref>
- 2022: The European Space Agency proposed a program called SOLARIS to operate Solar Power Satellites from 2030.<ref>Foust, Jeff (2022-08-19). "ESA to request funding for space-based solar power study". SpaceNews. Retrieved 2023-10-29.</ref>
- 2023: Caltech's Space Solar Power Demonstrator (SSPD-1) beams "detectable power" to Earth.<ref name=caltech2023edu/>
Non-typical configurations and architectural considerations
The typical reference system-of-systems involves a significant number (several thousand multi-gigawatt systems to service all or a significant portion of Earth's energy requirements) of individual satellites in GEO. The typical reference design for the individual satellite is in the 1-10 GW range and usually involves planar or concentrated solar photovoltaics (PV) as the energy collector / conversion. The most typical transmission designs are in the 1–10 GHz (2.45 or 5.8 GHz) RF band where there are minimum losses in the atmosphere. Materials for the satellites are sourced from, and manufactured on Earth and expected to be transported to LEO via re-usable rocket launch, and transported between LEO and GEO via chemical or electrical propulsion. In summary, the architecture choices are:
- Location = GEO
- Energy Collection = PV
- Satellite = Monolithic Structure
- Transmission = RF
- Materials & Manufacturing = Earth
- Installation = RLVs to LEO, Chemical to GEO
There are several interesting design variants from the reference system:
Alternate energy collection location: While GEO is most typical because of its advantages of nearness to Earth, simplified pointing and tracking, very small time in occultation, and scalability to meet all global demand several times over, other locations have been proposed:
- Sun Earth L1: Robert Kennedy III, Ken Roy & David Fields have proposed a variant of the L1 sunshade called "Dyson Dots"<ref>Kennedy, Robert G.; Roy, Kenneth I.; Fields, David E. (2013). "Dyson Dots: Changing the solar constant to a variable with photovoltaic lightsails". Acta Astronautica. 82 (2): 225–37. Bibcode:2013AcAau..82..225K. doi:10.1016/j.actaastro.2012.10.022.</ref> where a multi-terawatt primary collector would beam energy back to a series of LEO sun-synchronous receiver satellites. The much farther distance to Earth requires a correspondingly larger transmission aperture.
- Lunar surface: David Criswell has proposed using the Lunar surface itself as the collection medium, beaming power to the ground via a series of microwave reflectors in Earth Orbit. The chief advantage of this approach would be the ability to manufacture the solar collectors in-situ without the energy cost and complexity of launch. Disadvantages include the much longer distance, requiring larger transmission systems, the required "overbuild" to deal with the lunar night, and the difficulty of sufficient manufacturing and pointing of reflector satellites.<ref>"Lunarsolarpower". Archived from the original on 2016-05-26. Retrieved 2016-05-23.</ref>
- MEO: MEO systems have been proposed for in-space utilities and beam-power propulsion infrastructures. For example, see Royce Jones' paper.<ref>Royce Jones. "Beamed Energy In-Space Transportation System for Near Space Colonization" (PDF). Archived from the original (PDF) on 2016-06-10. Retrieved 2016-05-22.</ref>
- Highly elliptical orbits: Molniya, Tundra, or Quazi Zenith orbits have been proposed as early locations for niche markets, requiring less energy to access and providing good persistence.<ref>http://www.sspi.gatech.edu/welsom_isdc_reed.pdf Kevin Reed's QGSO proposal (Slide 25)</ref>
- Sun-sync LEO: In this near Polar Orbit, the satellites precess at a rate that allows them to always face the Sun as they rotate around Earth. This is an easy to access orbit requiring far less energy, and its proximity to Earth requires smaller (and therefore less massive) transmitting apertures. However disadvantages to this approach include having to constantly shift receiving stations, or storing energy for a burst transmission. This orbit is already crowded and has significant space debris.
- Equatorial LEO: Japan's SPS 2000 proposed an early demonstrator in equatorial LEO in which multiple equatorial participating nations could receive some power.<ref>"Space Future - SPS 2000 - an SPS Demonstrator".</ref>
- Earth's surface: Narayan Komerath has proposed a space power grid where excess energy from an existing grid or power plant on one side of the planet can be passed up to orbit, across to another satellite and down to receivers.<ref>Komerath, Narayanan. "The Space Power Grid: Synergy Between Space, Energy and Security Policies" (PDF). Georgia Tech. Retrieved December 4, 2022.</ref>
Energy collection: The most typical designs for solar power satellites include photovoltaics. These may be planar (and usually passively cooled), concentrated (and perhaps actively cooled). However, there are multiple interesting variants.
- Solar thermal: Proponents of solar thermal have proposed using concentrated heating to cause a state change in a fluid to extract energy via rotating machinery followed by cooling in radiators. Advantages of this method might include overall system mass (disputed), eliminating degradation due to solar-wind damage, and radiation tolerance. One recent thermal solar power satellite design by Keith Henson and others has been visualized here. Thermal Space Solar Power concept A related concept is here: Beamed Energy Bootstrapping The proposed radiators are thin wall platic tube filled with low pressure (2.4 kPa) and temperature (20 deg C) steam.
- Solar pumped laser: Japan has pursued a solar-pumped laser, where sunlight directly excites the lasing medium used to create the coherent beam to Earth.
- Stellaser: A hypothetical concept of a very large laser where a star provides both the lasing energy and the lasing medium, producing a steerable energy beam of unrivaled power.
- Fusion decay: This version of a power-satellite is not "solar". Rather, the vacuum of space is seen as a "feature not a bug" for traditional fusion. Per Paul Werbos, after fusion even neutral particles decay to charged particles which in a sufficiently large volume would allow direct conversion to current.[citation needed]
- Solar wind loop: Also called a Dyson–Harrop satellite. Here the satellite makes use not of the photons from the Sun but rather the charged particles in the solar wind which via electro-magnetic coupling generate a current in a large loop.
- Direct mirrors: Early concepts for direct mirror re-direction of light to planet Earth suffered from the problem that rays coming from the sun are not parallel but are expanding from a disk and so the size of the spot on the Earth is quite large. Lewis Fraas has explored an array of parabolic mirrors to augment existing solar arrays.<ref>Lewis M. Fraas. Self Pointing Mirror Satellites for Solar Power from Space (PDF). SSP Workshop Orlando FL, Dec 2015. Archived from the original (PDF) on 2016-07-01. Retrieved 2016-05-23.</ref>
Alternate satellite architecture: The typical satellite is a monolithic structure composed of a structural truss, one or more collectors, one or more transmitters, and occasionally primary and secondary reflectors. The entire structure may be gravity gradient stabilized. Alternative designs include:
- Swarms of smaller satellites: Some designs propose swarms of free-flying smaller satellites. This is the case with several laser designs, and appears to be the case with CALTECH's Flying Carpets.<ref>Silberg, Bob (April 6, 2016). "Will orbiting flying carpets light the world?". NASA.</ref> For RF designs, an engineering constraint is the thinned array problem.
- Free floating components: Solaren has proposed an alternative to the monolithic structure where the primary reflector and transmission reflector are free-flying.<ref>"Space-Based Storm Control". 17 April 2009.</ref>
- Spin stabilization: NASA explored a spin-stabilized thin film concept.
- Photonic laser thruster (PLT) stabilized structure: Young Bae has proposed that photon pressure may substitute for compressive members in large structures.<ref>Bae, Young (2007), "Photon Tether Formation Flight (PTFF) for Distributed and Fractionated Space Architectures", AIAA SPACE 2007 Conference & Exposition, American Institute of Aeronautics and Astronautics, doi:10.2514/6.2007-6084, ISBN 978-1-62410-016-1, retrieved 2022-05-10</ref>
Transmission: The most typical design for energy transmission is via an RF antenna at below 10 GHz to a rectenna on the ground. Controversy exists between the benefits of Klystrons, Gyrotrons, Magnetrons and solid state. Alternate transmission approaches include:
- Laser: Lasers offer the advantage of much lower cost and mass to first power, however there is controversy regarding benefits of efficiency. Lasers allow for much smaller transmitting and receiving apertures. However, a highly concentrated beam has eye-safety, fire safety, and weaponization concerns. Proponents believe they have answers to all these concerns. A laser-based approach must also find alternate ways of coping with clouds and precipitation.
- Atmospheric waveguide: Some have proposed it may be possible to use a short pulse laser to create an atmospheric waveguide through which concentrated microwaves could flow.<ref>Friedman, D. Clint (May 2009). "Electromagnetic (EM) Wave Attachment To Laser Plasma Filaments" (PDF).</ref><ref>Tzortzakis, Stelios; Couairon, Arnaud (26 February 2014). "A Waveguide Made of Hot Air". Physics. 7: 21. Bibcode:2014PhyOJ...7...21C. doi:10.1103/Physics.7.21.</ref><ref>"Events - "Long-lived Atmospheric Waveguide in the Wake of Laser Filaments"". phys.technion.ac.il. Archived from the original on 2017-02-16.</ref>
- Nuclear synthesis: Particle accelerators based in the inner solar system (whether in orbit or on a planet such as Mercury) could use solar energy to synthesize nuclear fuel from naturally occurring materials. While this would be highly inefficient using current technology (in terms of the amount of energy needed to manufacture the fuel compared to the amount of energy contained in the fuel) and would raise obvious nuclear safety issues, the basic technology upon which such an approach would rely on has been in use for decades, making this possibly the most reliable means of sending energy especially over very long distances - in particular, from the inner solar system to the outer solar system.
Materials and manufacturing: Typical designs make use of the developed industrial manufacturing system extant on Earth, and use Earth based materials both for the satellite and propellant. Variants include:
- Lunar materials: Designs exist for Solar Power Satellites that source >99% of materials from lunar regolith with very small inputs of "vitamins" from other locations. Using materials from the Moon is attractive because launch from the Moon is in theory far less complicated than from Earth. There is no atmosphere, and so components do not need to be packed tightly in an aeroshell and survive vibration, pressure and temperature loads. Launch may be via a magnetic mass driver and bypass the requirement to use propellant for launch entirely. Launch from the Moon the GEO also requires far less energy than from Earth's much deeper gravity well. Building all the solar power satellites to fully supply all the required energy for the entire planet requires less than one millionth of the mass of the Moon.
- Self-replication on the Moon: NASA explored a self-replicating factory on the Moon in the early 1980s.<ref>Replicating systems concepts: Self-replicating lunar factory and demonstration (Report). NASA. November 1, 1982. Retrieved January 31, 2023.</ref> More recently, Justin Lewis-Webber proposed a method of speciated manufacture of core elements<ref>Lewis-Weber, Justin (2016). "Lunar-Based Self-Replicating Solar Factory". New Space. 4 (1): 53–62. Bibcode:2016NewSp...4...53L. doi:10.1089/space.2015.0041.</ref> based upon John Mankins SPS-Alpha design.<ref>"ARTEMIS Innovation".</ref><ref>"NASA.gov" (PDF).</ref>
- Asteroidal materials: Some asteroids are thought to have even lower Delta-V to recover materials than the Moon, and some particular materials of interest such as metals may be more concentrated or easier to access.
- In-space/in-situ manufacturing: With the advent of in-space additive manufacturing, concepts such as SpiderFab might allow mass launch of raw materials for local extrusion.<ref>"Tethers Unlimited. SpiderFab Additive Manufacturing and Assembly On-Orbit". Archived from the original on 2016-05-19. Retrieved 2016-05-23.</ref>
Method of installation / Transportation of Material to Energy Collection Location: In the reference designs, component material is launched via well-understood chemical rockets (usually fully reusable launch systems) to LEO, after which either chemical or electrical propulsion is used to carry them to GEO. The desired characteristics for this system is very high mass-flow at low total cost. Alternate concepts include:
- Lunar chemical launch: ULA has recently showcased a concept for a fully re-usable chemical lander XEUS to move materials from the Lunar surface to LLO or GEO.<ref>George Sowers (15 December 2015). "Transportation Architecture for Cislunar Space" (PDF). United Launch Alliance. Archived from the original (PDF) on 2016-05-07. Retrieved 2016-05-23.</ref>
- Lunar mass driver: Launch of materials from the lunar surface using a system similar to an aircraft carrier electromagnetic catapult. An unexplored compact alternative would be the slingatron.
- Lunar space elevator: An equatorial or near-equatorial cable extends to and through the lagrange point. This is claimed by proponents to be lower in mass than a traditional mass driver.
- Space elevator: A ribbon of pure carbon nanotubes extends from its center of gravity in Geostationary orbit, allowing climbers to climb up to GEO. Problems with this include the material challenge of creating a ribbon of such length (36,000 km!) with adequate strength, management of collisions with satellites and space debris, and lightning.
- MEO Skyhook: As part of an AFRL study, Roger Lenard proposed a MEO Skyhook. It appears that a gravity gradient-stabilized tether with its center of mass in MEO can be constructed of available materials. The bottom of the skyhook is close to the atmosphere in a "non-keplerian orbit". A re-usable rocket can launch to match altitude and speed with the bottom of the tether which is in a non-keplerian orbit (travelling much slower than typical orbital speed). The payload is transferred and it climbs the cable. The cable itself is kept from de-orbiting via electric propulsion and/or electromagnetic effects.
- MAGLEV launch / StarTram: John Powell has a concept for a very high mass-flow system. In a first-gen system, built into a mountain, accelerates a payload through an evacuated MAGLEV track. A small on-board rocket circularizes the payload.<ref>"Startram - The Startram Project". Startram.</ref>
- Beamed energy launch: Kevin Parkin and Escape Dynamics both have concepts<ref>Parkin, Kevin L.G. (2006). The Microwave Thermal Thruster and Its Application to the Launch Problem (PhD). California Institute of Technology. doi:10.7907/T337-T709.</ref> for ground-based irradiation of a mono-propellant launch vehicle using RF energy. The RF energy is absorbed and directly heats the propellant not unlike in NERVA-style nuclear-thermal. LaserMotive has a concept for a laser-based approach.
In fiction
Space stations transmitting solar power have appeared in science-fiction works like Isaac Asimov's "Reason" (1941), that centers around the troubles caused by the robots operating the station. Asimov's short story "The Last Question" also features the use of SBSP to provide limitless energy for use on Earth.
Erc Kotani and John Maddox Roberts's 2000 novel The Legacy of Prometheus posits a race between several conglomerates to be the first to beam down a gigawatt of energy from a solar satellite in geosynchronous orbit.
In Ben Bova's novel PowerSat (2005), an entrepreneur strives to prove that his company's nearly completed power satellite and spaceplane (a means of getting maintenance crews to the satellite efficiently) are both safe and economically viable, while terrorists with ties to oil producing nations attempt to derail these attempts through subterfuge and sabotage.<ref>Bova, Ben (2006-10-31). Powersat. Macmillan. ISBN 0765348179.</ref>
Various aerospace companies have also showcased imaginative future solar power satellites in their corporate vision videos, including Boeing,<ref>https://www.youtube.com/watch?v=nEjPLHmFAM8 You Just Wait</ref> Lockheed Martin,<ref>https://www.youtube.com/watch?v=NQxfJzl2jkg The Next 100 Years</ref> and United Launch Alliance.<ref>https://www.youtube.com/watch?v=uxftPmpt7aA CIS-Lunar 1000</ref>
The solar satellite is one of three means of producing energy in the browser-based game OGame. The city building game SimCity 2000 also features a Microwave Power Plant.
In the 1978 anime TV series Future Boy Conan, SBSP enables the country of Industria to develop geomagnetic weapons, more powerful than nuclear weapons, that destroy entire continents.
See also

Lua error in mw.title.lua at line 318: bad argument #2 to 'title.new' (unrecognized namespace name 'Portal').
- Attitude control
- Climate engineering
- Friis transmission equation
- Future energy development
- Orbital station-keeping in GEO
- Lua error in Module:GetShortDescription at line 33: attempt to index field 'wikibase' (a nil value).
- Solar panels on spacecraft
- Solaris SBSP
- Space fountain – Extremely tall tower meant to launch spacecraft via pellets in vacuum tubes
- Space mirror
- Sun gun – Theoretical orbital weapon
- Znamya – Russian orbital mirror experiments in the 1990s
References
The National Space Society maintains an extensive space solar power library Archived 2018-04-14 at the Wayback Machine of all major historical documents and studies associated with space solar power, and major news articles Archived 2016-05-29 at the Wayback Machine.
External links
- European Space Agency (ESA) – Advanced Concepts Team, Space-based solar power
- William Maness on why alternative energy and power grids aren't good playmates and his plans for beaming solar power from space. in Seed (magazine)
- The World Needs Energy from Space Space-based solar technology is the key to the world's energy and environmental future, writes Peter E. Glaser, a pioneer of the technology.
- Reinventing the Solar Power Satellite", NASA 2004–212743, report by Geoffrey A. Landis of NASA Glenn Research Center
- Japan's plans for a solar power station in space - the Japanese government hopes to assemble a space-based solar array by 2040.
- Space Energy, Inc. - Space Energy, Inc.
- Whatever happened to solar power satellites? An article that covers the hurdles in the way of deploying a solar power satellite.
- Solar Power Satellite from Lunar and Asteroidal Materials Archived 2020-09-25 at the Wayback Machine Provides an overview of the technological and political developments needed to construct and utilize a multi-gigawatt power satellite. Also provides some perspective on the cost savings achieved by using extraterrestrial materials in the construction of the satellite.
- A renaissance for space solar power? by Jeff Foust, Monday, August 13, 2007 Reports on renewed institutional interest in SSP, and a lack of such interest in past decades.
- "Conceptual Study of A Solar Power Satellite, SPS 2000" Makoto Nagatomo, Susumu Sasaki and Yoshihiro Naruo
- Researchers Beam 'Space' Solar Power in Hawaii (Wired Science)
- [1] Archived 2018-04-14 at the Wayback Machine The National Space Society's Space Solar Power Library
- The future of Energy is on demand? Special Session at the 2010 Festival delle Città Impresa featuring John Mankins (Artemis Innovation Management Solutions LLC, USA), Nobuyuki Kaya (Kobe University, Japan), Sergio Garribba (Ministry of Economic Development, Italy), Lorenzo Fiori (Finmeccanica Group, Italy), Andrea Massa (University of Trento, Italy) and Vincenzo Gervasio (Consiglio Nazionale dell'Economia ed del Lavoro, Italy). White Paper- History of SPS Developpements International Union of Radio Science 2007
- International SunSat design competition
- A simulation of AM reception from an aerial powering two inductive loads and recharging a battery.
- De La Garza, Alejandro (June 1, 2023). "Scientists Just Got A Step Closer to The Sci-Fi Reality of Building Solar Power Stations in Space". Time. Archived from the original on June 5, 2023. Retrieved June 5, 2023.
Videos
- Solar power from space 5-minute video about space-based solar power plants by the European Space Agency
- Powering the Planet 20-minute streaming video from The Futures Channel that provides a "101" on space-based solar power
- Space Solar Power NewSpace 2010 Panel, 72 minutes
- Space Solar Power and Space Energy Systems SSI – Space Manufacturing 14 Panel – 2010 – 27 min
- NASA DVD in 16 Parts Exploring New Frontiers for Tomorrow's Energy Needs
- Space Solar Power Press Conference September 12, 2008 (71 minutes)[clarification needed][who?] National Space Society
- BBC One - Bang Goes the Theory, Series 6, Episode 5, Transmitting power without wires BBC/Lighthouse DEV Eye-safe Laser Based Power Beaming Demo
Lua error in Module:Authority_control at line 181: attempt to index field 'wikibase' (a nil value).